
Educational resources for practitioners, students, researchers and educators
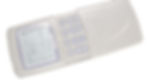
​
Neuromuscular Electrical Stimulation (NMES)
​
Navigation
​
Physiological vs Electrically Induced Contractions
Stimulation Parameters :
​
THIS IS THE FIRST OF 2 PAGES ABOUT NMES - THIS ONE COVERS THE GENERAL ISSUES AND PRINCIPLES - THE OTHER - LINK HERE - COVERS A RANGE OF CLINICAL APPLICATIONS
​
Overview
​
There is an increasing application of long term (i.e. chronic) electrical stimulation in order to modify or change muscle function. This work was initially concentrated around athlete strengthening and function, but in recent years, the intervention has crossed the boundary into clinical practice with an increasing range of applications. There are numerous studies that indicate that such stim is capable of changing muscle function parameters e.g. strength and endurance - and in fact it is potentially one of the most strongly evidenced interventions in the electrophysical realm - and yet, appears to be relatively under-utilised (compared with the strength and volume of the published research).
​
There are MANY different terms that are employed to describe this type of intervention, and it is suggested that a general term – like NeuroMuscular Electrical Stimulation (or NMES) is preferable to modality names based on specific machines.
​
Other terms - which will not be used hereafter - include :
Muscle Electrical Stimulation (MES)
Electrical Muscle Stimulation (EMS)
Neuro Muscular Stimulation (NMS)
Eutrophic Stimulation
. . . . and numerous others
​
Machines can be small, portable and battery powered, can be dedicated clinic units or indeed, NMES functions are available on almost all ‘multi-function’ electrotherapy machines, examples of which are illustrated below
​

The goal of NMES is to achieve a tetanic contraction (or at least a partial tetany) of muscle fibres in response to stimulation of motor nerves using surface electrodes. The primary reason for adopting the NMES terminology is that it is the motor nerve which is stimulated by the device - not the muscle itself. It is possible to stimulate a muscle directly - and if it is denervated that might be a therapy target - but this requires stimulation parameters which are usually not available on modern stimulators and will not be covered in the material on this page.
​
The mechanism of this intervention relates primarily to stimulation frequency, its intensity, the pulse duration and the 'pattern' by which it is delivered. Whilst there are multiple variables, the essentials of this application can be quite straightforward.
​
Muscle stimulation is not exclusive to NMES - it can be achieved with many other electrical stimulation devices - such as Interferential, Russian Stimulation or Burst Mode Alternating Currents (BMAC) - details of which are provided on the appropriate pages.
​
Electrically Induced vs Natural Muscle Contraction
​
Whilst the stimulation of a motor nerve will bring about an obvious muscle contraction, there is an ongoing controversy with regards how closely this might (or might not) mimic a 'natural' physiological contraction.
​
In a normal physiological contraction, the number of motor units which fire at any point in time is altered - increased force output is achieved with increased number of motor units activated. The Type I motor units will be the first to fire followed by the Type II fibres.
​
Essentially, the most significant difference is that an electrically evoked contraction is non-selective - it will recruit all fibre types in a synchronous fashion (so fast and slow motor units fire together), though it is argued that as the Type II motor fibres are larger, it may well be that the Type II motor units will fire earlier - which would be a complete reversal of the physiological pattern.
Whilst the detail of the differences are beyond the remit of this NMES page, Anthony (2020) and Bickel (2011) provide insightful analysis and evaluation of the current state of the art. Suffice it to say that the muscle contraction resulting from E Stim is NOT identical to a physiological contraction. Whether this is clinically meaningful has yet to be resolved - watch this space!
​
Early Research
​
There are a host of early studies which have informed this clinical application - some are animal studies whilst many involve healthy participants (typically sports students!).
​
Some of the critical early work (in my view) is that of Buller (1960) who demonstrated change in muscle behavious in response to switched (re-implanted) nerve supply. Fortunately Salmons and Vbrova (1969) demonstrated that this was possible by means of electrical stimulation.
​
In healthy participants, Hon Sun Loi (1988) demostrated the a 3 week period of stimulation resulted in significant strength gains - primarily in isometric strength, less so in concentric and not in eccentric. Stronger stimulation resulted in greater changes. The strength gains declined following the stimulation weeks - and there was also some crossover effect (strength gains in the non stimulated limb).
​
Balogun et al (1993) carried out similar work (but with 6 weeks stimulation) noting a 24% increase in strength in the stimulated limb and a 10% increase in the contralateral (non stimulated) limb. Thereby establishing that the effects of stim are not confined to local responses.
​
It is now recognised that the early changes (days / weeks) must have a NEURAL component - probably linked with spinal motor pool activation and synaptic facilitation, followed (medium to longer term) with fibre changes. Hortobagyi and Maffuletti (2011) provide a most insigtful analysis.
​
NMES Waveforms
​
Many manufacturers of NMES devices claim that THEIR waveform is 'special' and more effective than those provided by others - so what is the reality - based on the evidence??
​
At the end of the day, the waveform employed is NOT a critical issue in terms of clinical outcome. There is evidence (reviewed in Anthony, 2020 and Bickel et al, 2011 amongst others) that the biphasic asymmetric but balanced waveform is probably the more popular and probably the more effective. This would concur with my own reading of the available evidence. There are arguments that a biphasic symetrical waveform might be more effective when it comes to stimulation of large muscle groups (e.g. the quads), but it might actually be more to do with the phase charge than the waveform per se.
​
At the end of the day, if the device offers a choice between stimulating waveforms, then an asymmetric biphasic, balanced (zero net DC) is probably the one to go for.
​
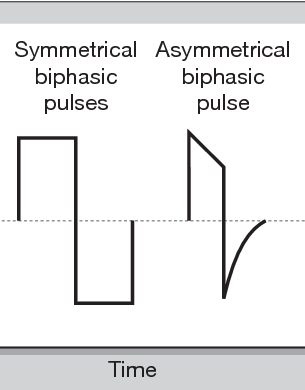
after semanticscholar.org
Kramer et al (1984), Walmsley et al (1984), Snyder-Mackler et al 1989) have all published evidence which supports the asymmetric over the symmetric waveform (max quads force production).
There is an approximately linear relationship between CURRENT INTENSITY and FORCE OF CONTRACTION (Ferguson et al 1989, Underwood et al 1990). The greatest effects with least current intensity by using BIPHASIC PULSED or BURST AC currents. Recent work by Ward et al (2006-2008) lends some support to the use of burst AC (medium frequency – Russian Stim, Aussie Stim) stimulation, though there remains some controversy, yet to be resolved.
Stimulation Patameters
​
In addition to the waveform consideration, there are several other parameters which merit consideration, and which will likely influence the outcome of the stimulation. Detailed considerations are found in the current literature. This summary information is based on my interpretation of that literature.
​
Stimulation Intensity
This is best managed on a subjective rather than an objective basis.
As with TENS, Interferential and other forms of stimulation, it is not possible to say that it should be used at XxX or YyY mA stimulation intensity or that you need a particular current for a specific muscle group.
It is necessary to stimulate at a level sufficient to achieve a 'good' muscle contraction. The most commonly used method to achieve this is to gradually increase the stimulation intensity (with the patient making no contribution) untill a significant contract is achieved with the machine alone. Once this level is established, the patient is instructed to 'join in' with the stimulation patters, which is straightforward if a patterned / ramped stimulation (see below) is employed.
In research and highly specific sports rehabilitation programmes, the 'amount' of muscle contraction can be related to %MVIC (using a dynanometer or Cybex type system). Some of the clinical research papers cited in the reference list will provide details if this is a relevant application system. It is probably unreaslistic - and unnecessary - in routine clinical application.
​
Duty Cycle : (ON : OFF ratio)
In NMES application, it is important to consider the proportion of the time during which the muscle is working (stimulated) against the amount of time for which it is rested. This is managed (all machines) via some kind of Duty Cycle facility. This determines the absolute and relative proportions of the total treatment period for work and rest.
​
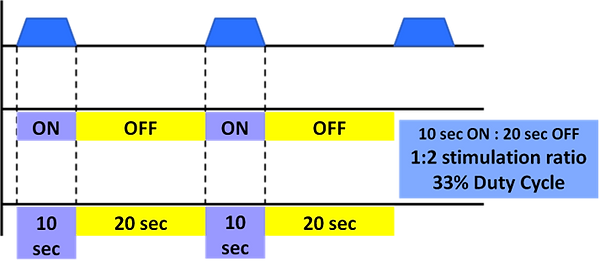
Example of a 10 sec stimulation followed by a 20 sec rest (which equates to a 33% duty cycle)
It is suggested that the minimum is to use equal cycles (1:1) but only for the stronger / end rehab / fit patients - this would not be approprate for those with significant strength loss / weakness / atrophy
​
Using longer rest periods will reduce the potential for fatigue - and therefore, as a general principle, the weaker the muscle being stimulated, the longer the rest phase (higher proportion rest)
​
If one employed a duty cycle of 33%, the rest period(s) would be 2 x longer than the stimulation periods - giving the muscle adequate rest before the next contraction.
​
One might start at 1:9 for patients with very weak musculature and/or severe atrophy and progressively reduce (towards 1:1) as the muscle state improves.
​
For example, if using stim for quads in a very weak patient (post TKA) you might use a 1:9 ratio, so 10 sec stim would be followed by 90 sec rest.
​
Some machines allow control over this function by setting a duty cycle % whilst others allow it to be managed by setting the ACTUAL stimulation time and the actual rest time.
Ramp
​
The RAMP setting (available on most, if not all stimulators, makes the stimulation more comfortable, closer to a physiological contraction and also makes it easier for the user to join in (add voluntary effort) to the stimulated contraction - whether that is for the quads post knee surgery or to the pelvic floor for a patient with continence issues.
​
Ramping is divided into RAMP UP and RAMP DOWN phases - identified in the image below
​
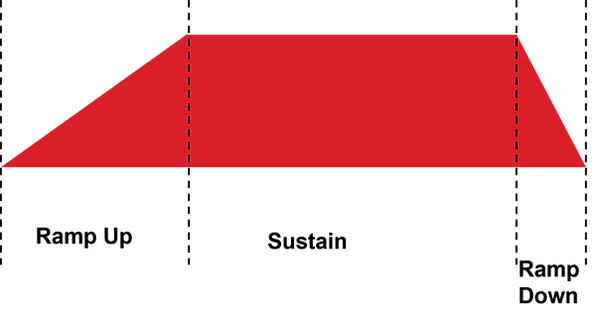
It can be seen that by employing both ramp UP and DOWN, the stimulation is gradually increased at the start of the contraction and gradually released at the end. It is common to employ a LONGER RAMP UP (typically 2 - 4 seconds) and a SHORTER RAMP DOWN (ytypically 1 - 2 seconds) though these values are certainly not 'fixed' in any way
A visual representation of a 10 second stimulation including ramp up and ramp down - as might typically be utilised is illustrated below
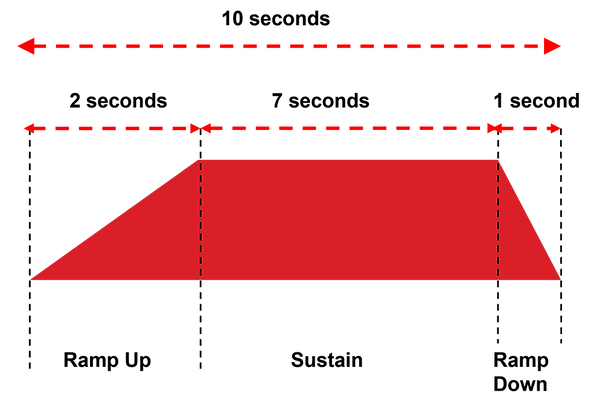
Pulse Duration
​
Whereas TENS gets its best results with short duration pulses, when it comes to NMES, longer pulses (relatively) appear to be more effective. Reading through the research, there are a wide range of pulse durations that have been used in the research - and to some extent or another, they all work.
Given a choice (most, but not all machines offer choice), the pulse duration in the range of 350 - 450 microsec is probably the optimal choice
​
Gorgey et al (2006) are among several who have investigated pulse duration effects on muscle output - comparing a range from 150 - 450 microsec - and there was no doubt that the longer duration pulses generated more muscle activity and increased force generation.
​
In days gone by, pulses were even longer - going up into the millisecond range - but these are employed far less often in modern devices. There is possibly still a place for them - but I will leave that debate to one side for the moment on the basis that your modern stimulator is unlikely to offer you such an option
​
Pulse Frequency
​
There is a pretty straightforwad relationship between the stimulation frequency and the amount of muscle torque developed (higher frequency, more torque)
​
The obvious thing to do then is to stimulate at a higher frequency . . . . the problem being that fatigue also increases as the frequency goes up. This is less of an issue in ultra fit and able sports people - but a patient population, who are rather less likely to be ultra fit in the first place, these higher frequencies (over 50Hz) are far less commonly used
​
Looking at the clinical research, frequencies between 10 and 50 Hz are most commonly used - the compromise between how much muscle activation is achieved vs fatigue and discomfort
​
In clinical guidelines on the topic (e.g. Nussbaum et al 2017), no matter whether the application is shoulder subluxation post stroke, muscle management in intensive care or musculoskeletal rehab post injury / surgery, the frequencies beteen 30 and 50Hz dominate the evidences recommendations.
​
Electrodes
​
Lastly on the 'variable' front (though of course they are not really a variable) there comes the question of electrode - both type and positioning
​
Most commonly in modern therapy practice, pre-gelled, self adhesive electrodes are widely used - though it is appreciate that in come countries, the use of carbon-rubber electrodes is still the normal.
​
Generally larger electrodes will be (a) more comfortable and (b) more effective. There have been numerous studies looking at this, but the paper by Alon et al (1994) provides clear evidence for this general rule of thumb. Smaller electrodes are certainly less comfortable, and if the user is unable to tolerate the required level of stimulation due to pain / discomfort, and therefore never reaches a high enough stimulation to achieve an adequate contraction, then the treatment is effectively a waste of time. In addition to the Alon paper, Anthony (2020) provides a useful analysis regarding electrode size for those who are interested.
​
​
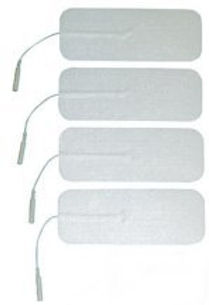


Examples of pre-gelled and carbon rubber electrodes for NMES
Electrode Positioning
​
There is a lot of complexity propogated by some when it comes to electrode positioning. Whist it is appreciated that there are some complex situations, there is a fairly simple solution - and one that will be effective in most, if not all clinical situations
​
Please one electrode at either end of the muscle / muscle group belly
​
Some advocate the positioning of one electrode over the motor point of the muscle of interest. Well, as can clearly been seen from the evidence, the motor points are NOT in a fixed position. For those of you who spent HOURS learning the (apparent) positions of all the motor points, you will be disappointed to know that they are in fact pretty variable in their relative position. Any text which includes motor point maps will simply be representing the average kind of position in which they can be found. The study by Botter et al (2011) illustrates the variability of the motor point locations across a group of individuals as the figure below illustrates
​
​
​
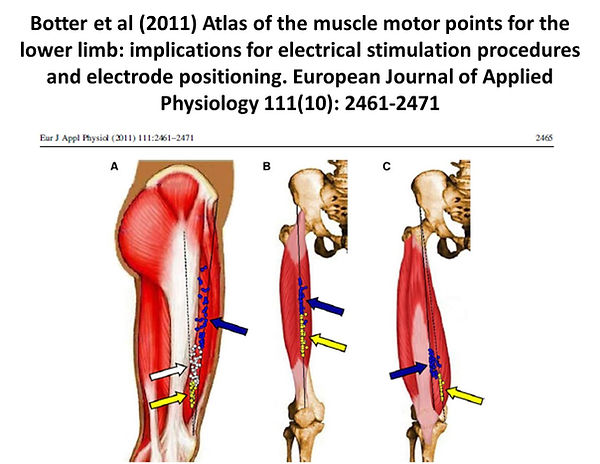
It is also argued that the red/black lead orientation makes a different - and that the negative (black) lead would ideally be placed at the motor point. Whilst there are technical (small) differences in electrode orientation, in clinical reality, especially when using a biphasic waveform, it makes no real difference to the effective outcome - so you can safely ignore the red/black anode/cathode lead argument
​
As a final comment on electrodes, by way of confirming the obvious, the SHAPE of the electrodes make no difference to the outcome - so square, rectangular, circular or oval - are all equally effective
THERE IS A SECOND PAGE ALL ABOUT CLINICAL APPLICATION - EITHER SELECT FROM THE MODALITIES MENU OR FOLLOW THIS LINK
References
​
There is a chapter in the new edition of the textbook which covers this material
Anthony, J. (2020) Chapter 16 : Neuromuscular Electrical Stimulation
IN: Watson, T. and Nussbaum, E. Electrophysical Agents: Evidence Based Practice. Elsevier
​
​
References cited on this page :
​
Alon, G. et al (1994) Effects of electrode size on basic excitatory responses and on selected stimulus parameters Journal of Orthopaedic and Sports Physical Therapy 20(1): 29
Balogun, J. A. et al (1993) High voltage electrical stimulation in the augmentation of muscle strength: effects of pulse frequency Arch Phys Med Rehabil 74(9): 910
Bickel, C. et al (2011) Motor unit recruitment during neuromuscular electrical stimulation: a critical appraisal European Journal of Applied Physiology 111(10): 2399
Botter, A. et al (2011) Atlas of the muscle motor points for the lower limb: implications for electrical stimulation procedures and electrode positioning Eur J Appl Physiol 111(10): 2461
Buller, A. J. et al (1960) INTERACTIONS BETWEEN MOTONEURONES AND MUSCLES IN RESPECT OF THE CHARACTERISTIC SPEEDS OF THEIR RESPONSES Journal of Physiology 150: 417
Gorgey, A. S. et al (2006) Effects of neuromuscular electrical stimulation parameters on specific tension Eur J Appl Physiol 97(6): 737
Hortobágyi, T.N. Maffiuletti (2011) Neural adaptations to electrical stimulation strength training European Journal of Applied Physiology 111(10): 2439
Kramer, J. et al (1984) Comparison of voluntary and electrical stimulation contraction torques Journal of Orthopaedic & Sports Physical Therapy 5(6): 324
Nussbaum, E. L. et al (2017) Neuromuscular Electrical Stimulation for Treatment of Muscle Impairment: Critical Review and Recommendations for Clinical Practice Physiother Can 69(5): 1
Salmons, S.G. Vrbova (1967) Changes in the speed of mammalian fast muscle following longterm stimulation J Physiol 192(2): 39P
Salmons, S.G. Vrbová (1969) The influence of activity on some contractile characteristics of mammalian fast and slow muscles J Physiol 201(3): 535
Snyder-Mackler, L. et al (1989) A comparison of torque generating capabilities of three different electrical stimulating currents J Orthop Sports Phys Ther 10(8): 297
Walmsley, R. et al (1984) A comparison of torque generated by knee extension with a maximal voluntary muscle contraction vis-a-vis electrical stimulation Journal of Orthopaedic & Sports Physical Therapy 6(1): 10
​